I have decided to get this section of my lab page going again after a very busy year of moving my lab to UC Berkeley and becoming a parent. As a first pass, I’ve decided to collapse my tree diseases tab and Blog tab into one. So here are two old posts that need a new home (I was unable to move the comments, so I apologize for losing those):
Tree disease update: Ash dieback (published Dec 26, 2012)

If you’re living in the UK or elsewhere in Europe, then you’ve already heard about the scary new disease of ash trees, known here as Ash dieback or in France as “Chalarose.” Like many tree diseases that have devastated forests, this one is caused by a fungus, Hymenoscyphus pseudoalbidus (anamorph: Chalara fraxinea [1]). According to the Forestry Commission, the fungus was introduced in February 2012 by import of infected trees from a nursery in the Netherlands to one in Buckinghamshire. It has already caused serious problems in Poland (where 3,000 infected trees fell on one windy night), Denmark (killing 95% of their trees), Lithuania (resulting in mortality of 60% of all ash stands), Austria, Belgium, the Czech Republic, Finland, France, Germany, Hungary, Italy, the Netherlands, Norway, Slovenia and Sweden, and is now wreaking havoc across the UK (Map of confirmed infections). This is very bad news indeed for our woodlands, as ash trees make up over 10% of the trees we have.
As a scientist, when I first hear about an emerging disease the questions I want answers to are: 1) Where did it come from? 2) How is it transmitted? 3) Why is it becoming a problem now? 4) What are the symptoms? And 5) Is there any natural variation in susceptibility? So, after surfing around on the web and reading some recent papers, here is what we know so far:
1) Where did it come from? As with many emerging diseases, the answer to this is not clear. The pathogen is closely related to another non-pathogenic fungus, Hymenoscyphus albidus, which has been widespread in Europe since the mid 19th century. (Interestingly, it seems as though the spread of the pathogenic species has lead to local extinctions of the native fungus in parts of Denmark [2].) The fungus was first described in 2006, but seems to have been around since the early 90s, when it devastated forests in Poland. Whether the species is a mutant of the closely related, yet harmless, H. albidus remains to be seen. As we are now in the genome era, the most informative way of determining how this new species came to be is to take a comparative genomic approach. For many pathogens, comparing their whole genome to those of closely related, but non-pathogenic species has allowed researchers both to infer the phylogeny of the pathogen and to identify regions of the genome that are likely to be involved in making it harmful to its host. This approach has been successfully used to identify candidate genes associated with virulence in a number of Pseudomonas syringae bacterialpathogens [3] and to demonstrate the movement of genes from pathogens to free-living bacteria[4].
Comparative genomics can also help elucidate whether the pathogen is truly newly emerged (which you could conclude if there was very little genetic variation among the isolates collected from different parts of the species range) or whether it has been around for a while. Surprisingly, there is evidence from molecular characterization of strains from Finland, Estonia and Latvia that there is quite a bit of variation already, suggesting that this fungal species has either been around for quite some time already or has evolved multiple times from a closely related species [5]. For now, we will have to wait and see what story the H. pseudoalbidus genome has to tell us.
2) How is it transmitted? The latest headlines from BBC and the Guardian state that the number of sightings has doubled in the last month, with about 300 confirmed cases in the UK. (Of course, what they don’t emphasize is that the awareness of the disease has increased exponentially since the news broke that the first case had been found back in early November.) So how is it moving around? And how is it infecting new trees once it arrives? It seems that the sexual stage is wind dispersed, with a suggested dispersal distance of 20 to 30 km per year.
The best way to determine how a disease is transmitted is by having lots of data over multiple years and then building a series of models that predict how the spread would look under varying assumptions – e.g. if it is limited by the dispersal rate of a vector, such as an insect, or the wind – and then testing which model fit the actual spread the best. Given the speed at which this pathogen has spread across Europe, it seems that wind dispersal is the most likely explanation for spread within forests and that human transport of saplings from country to country is the most likely explanation for new epidemics. It’s unclear exactly how the pathogen infects new trees once it gets to a new place, but there is some evidence it is through the leaves (this is true of many plant pathogens).
3) Why is it becoming a problem now? Again, the short answer is that we don’t know. The longer answer is that it may be related to our colder, damper summers over the past few years or it may be related to tree stress.
4) What are the symptoms? A little video from the Forestry commission on how to identify the symptoms of ash dieback:
5) Is there any natural variation in susceptibility? The good news is that there is some burgeoning evidence for genetic resistance to the disease, as not all trees die of infection. A study of trees in Denmark found that individuals with early leaf senescence (i.e., those that lost their leaves early in the season) were less susceptible than late-senescing individuals [6]. Interestingly, early leaf loss is also a symptom of the disease. Unfortunately, it looks like only a very small proportion of trees are resistant [7]; most likely too few to prevent a severe population crash. However, the presence of resistance at all suggests that evolution can work its magic. That being said, natural selection can be a painfully slow process, especially for such a long-lived organism. Therefore, a little bit of artificial selection (where individuals that are known to be resistant are crossed and their offspring are planted) could be a good solution to slowing the spread of the disease and eventual eradication.
Finally, let’s not forget that it’s not just the ash trees that are under threat! These trees are host to about 30% of all UK lichens, many of which are currently considered endangered species, and any number of bacterial species [8]. Also, there are at least two moth species that specialize on ash: the Dusky thorn (Ennomos fuscantaria) the centre-barred sallow (Atethmia centrago). This disease therefore has the potential to be a real threat of local ecosystems if left unchecked.
For more information:
1) If, like me, you have no idea what an anamorph is, it turns out the species was described twice in two different stages of its life cycle: the sexual stage – the teleomorph, and the asexual stage – the anamorph. The former is usually a fruiting body while the latter is more mold-like, which explains why they might have been considered different species.
2) McKinney, L., Thomsen, I., Kjær, E., Bengtsson, S., & Nielsen, L. (2012). Rapid invasion by an aggressive pathogenic fungus (Hymenoscyphus pseudoalbidus) replaces a native decomposer (Hymenoscyphus albidus): a case of local cryptic extinction? Fungal Ecology, 5 (6), 663-669 DOI: 10.1016/j.funeco.2012.05.004.
3) Baltrus, David A., et al. “Dynamic evolution of pathogenicity revealed by sequencing and comparative genomics of 19 Pseudomonas syringae isolates.” PLoS pathogens 7.7 (2011): e1002132.
4) Ma, Li-Jun, et al. “Comparative genomics reveals mobile pathogenicity chromosomes in Fusarium.” Nature 464.7287 (2010): 367-373.
5) Rytkonen, A., Lilja, A., Drenkan, R., Gaitner, T., and Hantula, J. 2010. First record of Chalara fraxinea in Finland and genetic variation among isolates sampled from Åland, mainland Finland, Estonia, and Latvia. For. Path.
6) McKinney, Lea Vig, et al. “Genetic resistance to Hymenoscyphus pseudoalbidus limits fungal growth and symptom occurrence in Fraxinus excelsior.” Forest Pathology 42.1 (2011): 69-74.
7) McKinney, Lea Vig, et al. “Presence of natural genetic resistance in Fraxinus excelsior (Oleraceae) to Chalara fraxinea (Ascomycota): an emerging infectious disease.” Heredity 106.5 (2010): 788-797.
8) Ellis, Christopher J., Brian J. Coppins, and Peter M. Hollingsworth. “Tree fungus: Lichens under threat from ash dieback.” Nature 491.7426 (2012): 672-672.
***********************************************************************
Why I study tree diseases: (published Nov 26, 2012)
About 5 years ago this month, my research took a very interesting twist. When I arrived at Oxford as an NSF international research fellow with a plan to work on bacteria and phages in tomato plants, I discovered (thanks to conversations with the amazing Gail Preston) that the same bacterial species causing the disease I was interested in, Pseudomonas syringae, was also killing all of the horse chestnut trees in Britain.My interest in this amazing pathogen stemmed from work published in 2007 by Maelli Melotto in which she discovered two incredible facts that exemplify the coevolutionary process. First, she and her coauthors found that when plants recognise bacteria growing on their leaves they close their stomata (the pores on the leaf surface) to stop the bacteria invading the leaf interior. As if that’s not cool enough, certain bacterial species – such as P. syringe – have figured out a way to mimic the hormone used by the plant to “trick” it into thinking the bacterium is no longer there, and to reopen it’s stomata. At that point, these motile bacterial cells swarm through the stomatal opening straight into the leaf and can wreak havoc on the host [1].
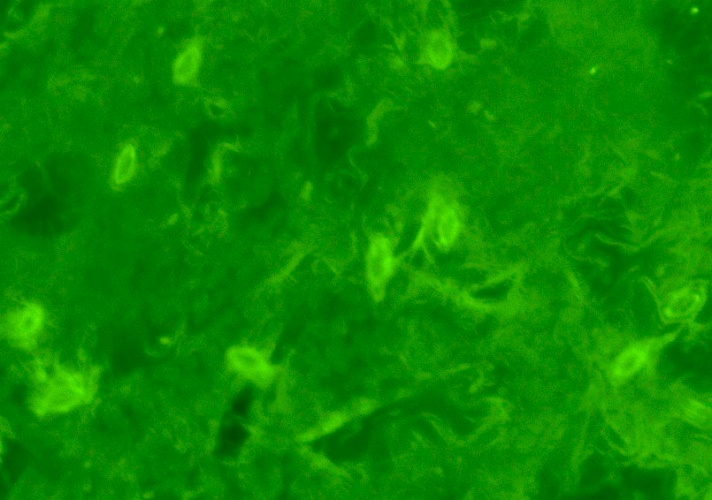
So back to trees. There is a new and particularly harmful strain of P. syringae that has evolved to infect horse chestnut trees [see Forest Research’s info page here]. We still don’t know where it came from, how it is transmitted from tree to tree, or even how it enters its host. But we do know that this bacterium is highly virulent and is infecting over half the horse chestnut trees in Britain [2]. Recent work in which they compared the whole genome of this bacterium to that of other P. syringaes suggests that it is very closely related to the bacterium that caused leaf spots on Indian horse chestnut in India in 1969, but also that it has evolved since then and expanded rapidly [3]. Bleeding canker first appeared near Wimbledon in the year 2000, and has already spread as far as northern Scotland, Wales, and Ireland. It has even made it to Cornwall – which is no small feat. So why is this pathogen spreading so rapidly? And might the reason for its increasing spread also explain the myriad of other tree diseases making headline news (Sudden Oak Death, Acute Oak Decline, Ash Dieback)? The causal agents aren’t the same – some are caused by fungi, others by bacteria, and others still by insect pests – but could there be an underlying cause for all of these threats to tree health? Only time (and research) will tell! So stay tuned.

Among the many possibilities for the recent increase in tree disease is the rapidly changing climate. Another possibility is that, while trees are able to fight off one or a few of these pests, the constant introduction of new pests into the environment is overwhelming the trees immune system. For example, horse chestnut trees are threatened both by a leaf-mining moth and the bacterium, P. syringae, and our poor oak trees are fighting against beetles, fungi and bacteria! And yet another possibility is that trees just can’t evolve quickly enough to keep up with all of the pests and pathogens we are introducing by moving species around the world. Trees often live for 100s of years, and take years before they begin reproduction. This makes the timescale for the evolution of tree populations quite long – especially when you compare it to the rapidly evolving populations of their antagonists, with generations of days or even hours. How are they to keep up? So now you have an idea of the big questions keeping me up at night. Hopefully my upcoming work with, among others, Jessica Metcalf and Roosa Leimu at Oxford will help gain insight into these issues and secure a place for our tree houses for years to come.

1) Melotto M, Underwood W, Koczan J, He SY. 2006. Plant stomata function in innate immunity against bacterial invasion. Cell 126:969-980. 2) Report on the National Survey to Assess the Presence of Bleeding Canker of Horse Chestnut Trees in Great Britain. Forestry Commission. http://www.forestry.gov.uk/pdf/bleedcankersurveyrep020408.pdf/$FILE/bleedcankersurveyrep020408.pdf 3) Green, S., Studholme, D. J., Laue, B. E., Dorati, F., Lovell, H., Arnold, D., … & Kamoun, S. (2010). Comparative genome analysis provides insights into the evolution and adaptation of Pseudomonas syringae pv. aesculi on Aesculus hippocastanum. PLoS One, 5(4), e10224.